What is Quantum Computing?
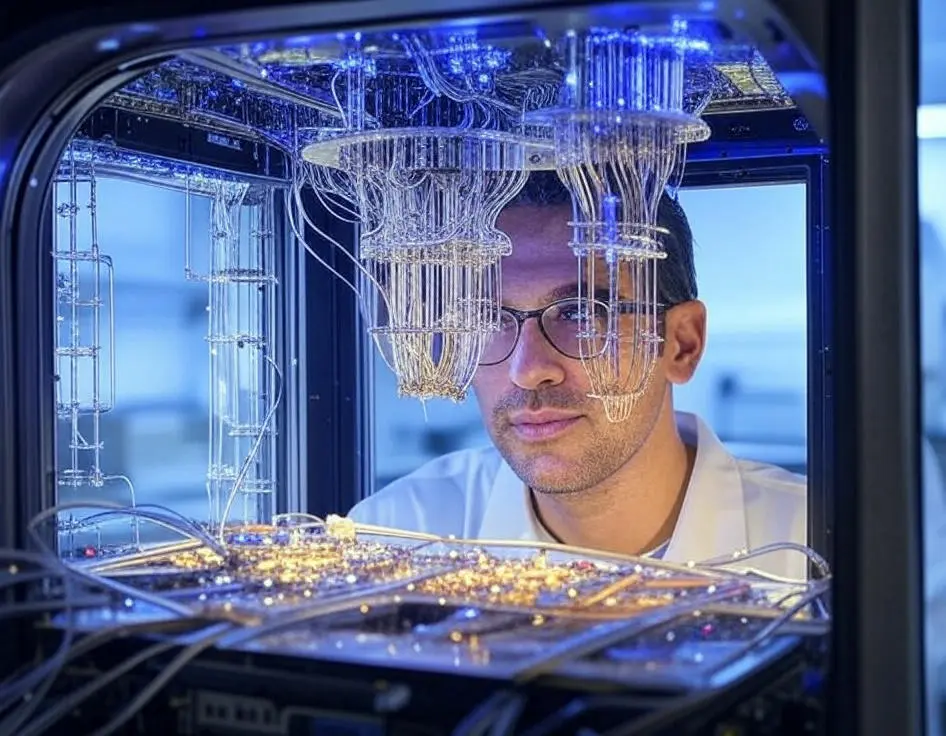
Quantum computing is a new way of computing that uses the principles of quantum mechanics, a field of physics dealing with very small particles. Instead of using traditional bits (0 or 1), it uses quantum bits, or qubits, which can be 0, 1, or both at the same time. This ability, called superposition, lets quantum computers handle many calculations at once, which could be much faster for certain tasks.
Table of Content
Key Concepts
- Superposition: Qubits can exist in multiple states, like spinning a coin that’s both heads and tails until you look at it. This allows for parallel processing.
- Entanglement: Qubits can be linked so that the state of one instantly influences another, even if far apart, enhancing computation coordination.
- Quantum Interference: Algorithms can boost correct answers and cancel out wrong ones, improving efficiency.
Potential Applications
Quantum computing could revolutionize fields like:
- Breaking encryption codes, impacting cybersecurity.
- Simulating molecules for faster drug discovery, aiding medical research.
- Optimizing complex systems, such as traffic flow or supply chains, for better efficiency.
Current State
As of March 2025, quantum computing is still in its early stages. While progress is rapid, challenges like quantum noise and error correction mean it’s not yet practical for everyday use. However, 2025 is seen as a pivotal year, with expectations of significant breakthroughs, especially in scaling up qubits and improving stability.
A Comprehensive Overview of Quantum Computing
Quantum computing represents a transformative approach to computation, leveraging the principles of quantum mechanics to process information in ways that differ fundamentally from classical computing. This survey note aims to provide a detailed exploration of quantum computing, covering its definition, key concepts, potential applications, and current state, with a focus on developments in 2025. It includes all relevant details from recent analyses, ensuring a thorough understanding for both novices and those with a technical background.
Definition and Foundations
Quantum computing is defined as a computing paradigm that exploits quantum mechanical phenomena, such as superposition and entanglement, to perform operations on data. Unlike classical computers, which rely on bits that are either 0 or 1, quantum computers use quantum bits, or qubits, which can exist in a superposition of states. This means a qubit can be 0, 1, or a combination of both simultaneously, enabling the evaluation of multiple input values at once, a concept known as quantum parallelism.
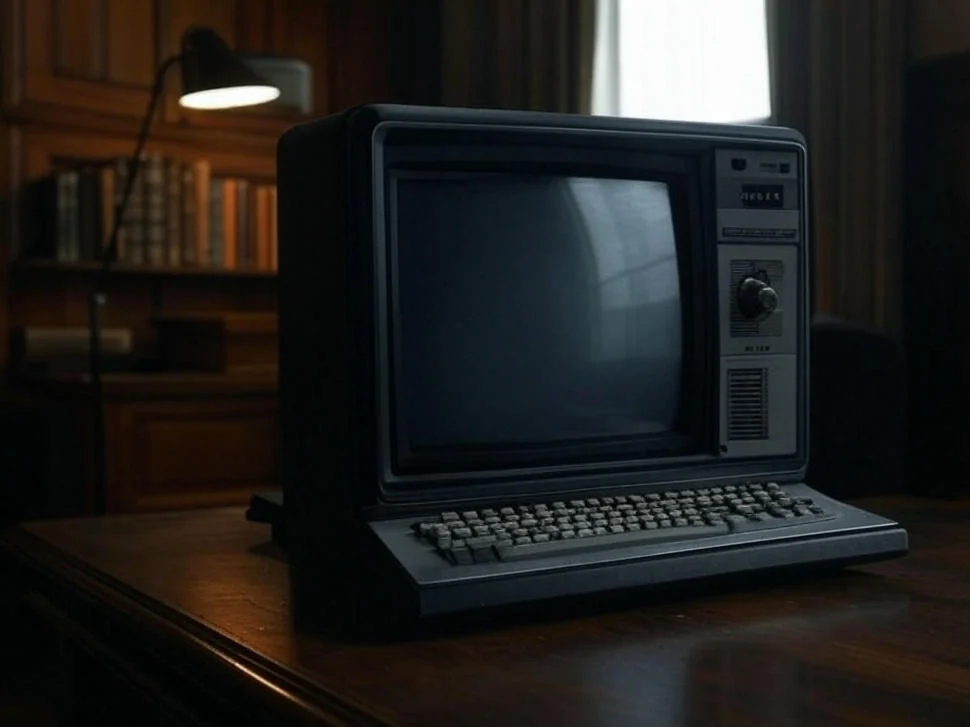
The field emerged from theoretical work in the 1970s, with significant milestones including Richard Feynman’s 1982 suggestion that quantum systems could simulate other quantum systems more efficiently than classical computers. This led to the development of quantum algorithms, such as Shor’s algorithm for factoring large numbers and Grover’s algorithm for searching unsorted databases, both of which offer potential speedups over classical methods.
Key Concepts and Technical Details
The following table summarizes the core concepts of quantum computing, drawn from recent authoritative sources:
Concept | Description | Key Implications |
Qubit | Basic unit of quantum information, analogous to a classical bit, but can be in superposition of states (${ | 0\rangle}$ and ${ |
Superposition | State where a qubit is a linear combination of basis states, e.g., ${ | ψ\rangle} = α |
Entanglement | Correlation between qubits where the state of one instantly influences another, even at a distance. | Enhances computation by coordinating operations, used in algorithms like quantum teleportation. |
Quantum Interference | Manipulation of probability amplitudes to amplify correct solutions and cancel incorrect ones. | Central to quantum algorithms, enabling efficient problem-solving through constructive and destructive interference. |
Quantum Decoherence | Loss of quantum coherence due to environmental interaction, introducing noise. | Major challenge; requires isolation and error correction to maintain qubit stability. |
Quantum Error Correction (QEC) | Methods to suppress errors, allowing computations longer than decoherence time if error rate is low (e.g., 10⁻³ for depolarizing noise). | Increases qubit count needed; for 1000-bit factoring, may require 10⁷ qubits with correction. |
Universal Gate Set | Set of gates (e.g., all single-qubit gates and CNOT) enabling any quantum computation, per Solovay-Kitaev theorem. | Allows representation of any unitary operation, essential for programmability. |
DiVincenzo’s Criteria | Requirements for practical quantum computers: scalable qubits, initializable states, fast gates vs. decoherence, universal gate set, readable qubits. | Critical for engineering; listed by David DiVincenzo, guiding current research efforts. |
These concepts are supported by recent research, such as the Quantum Algorithm Zoo (Quantum Algorithm Zoo), archived in 2022, which catalogs algorithms demonstrating quantum advantages.
Potential Applications and Use Cases
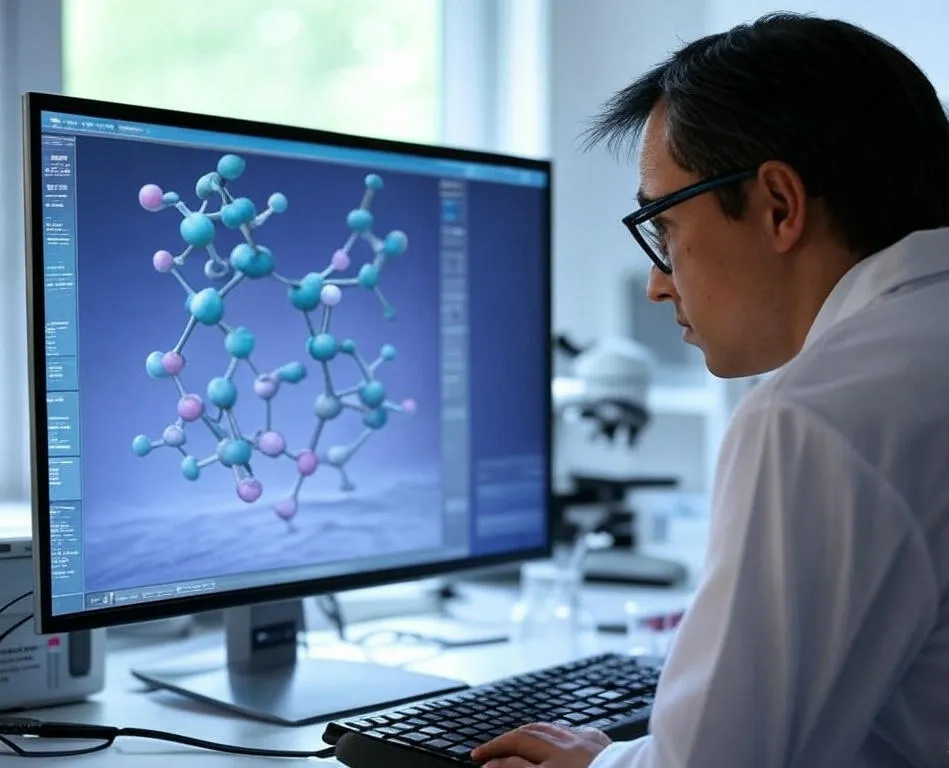
Quantum computing holds promise for solving problems intractable for classical computers, with applications spanning multiple domains:
- Cryptography: Quantum computers could break widely used encryption schemes, such as RSA, using Shor’s algorithm, prompting the development of quantum-resistant cryptography. This has significant implications for cybersecurity, as noted in recent analyses (MIT Technology Review: What’s next for quantum computing).
- Drug Discovery: Simulating molecular interactions at the quantum level could accelerate drug development, as quantum computers can model complex chemical systems more accurately. This is highlighted in recent studies on quantum chemistry applications (Open Access Government: Latest developments in quantum computing).
- Optimization Problems: Quantum algorithms could optimize complex systems, such as traffic flow, supply chains, and financial portfolios, offering efficiency gains. For example, Grover’s algorithm could search unsorted databases quadratically faster, impacting logistics and AI.
- Material Science: Designing new materials with desired properties, such as superconductors or lightweight alloys, could be expedited through quantum simulations, as noted in recent DOE reports.
An unexpected detail is the integration of machine learning with quantum systems, such as frameworks like TorchQC, which enhance computational power for quantum dynamics and control, potentially optimizing algorithms for real-world scenarios.
Current State and Developments in 2025
In 2025, quantum computing is in the noisy intermediate-scale quantum (NISQ) era, characterized by systems with up to a few hundred qubits but prone to errors due to decoherence and noise. Recent reports indicate significant progress, with expectations of major advancements in 2025, driven by global initiatives and technological breakthroughs.
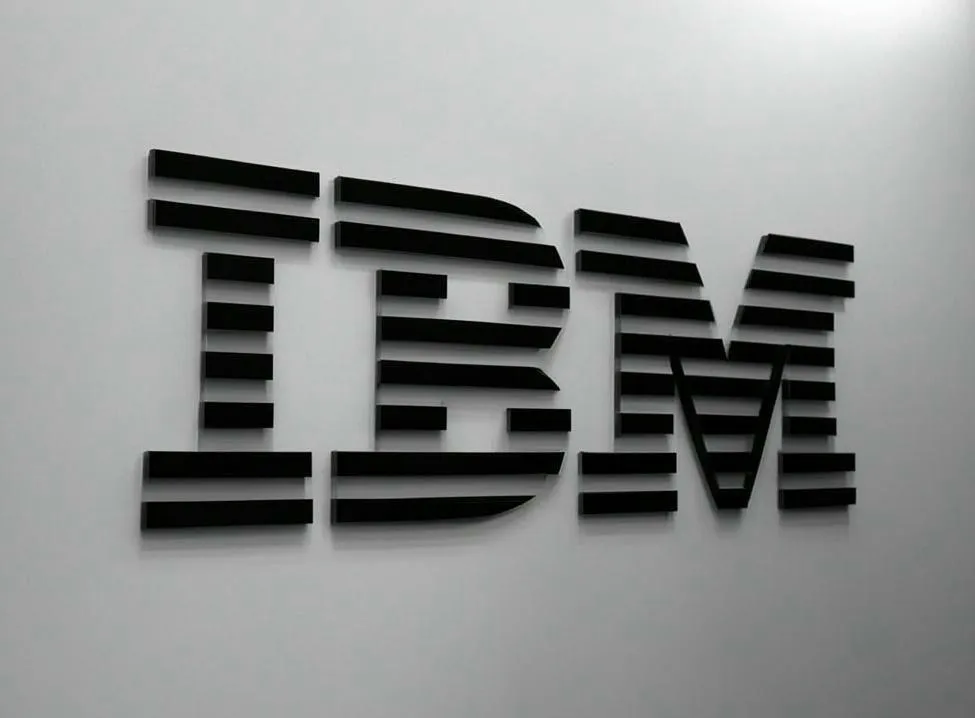
- Qubit Scaling: IBM plans to release a quantum computer with over 400 qubits in 2025, aiming to hit new records by linking multiple chips, as reported by new scientist. SpinQ claims a 1121-qubit system, though verification is ongoing (SpinQuanta: Discover the World’s Largest Quantum Computer in 2025).
- Quantum Error Correction (QEC): The field is entering the QEC era, with nearly two-thirds of quantum hardware companies prioritizing real-time error correction, essential for scaling and practical applications, as noted in The Quantum Insider.
- International Year of Quantum Science and Technology: The United Nations has designated 2025 as this, reflecting global recognition of its potential, with increased investments and research, as reported by CIO.
Challenges remain, including maintaining qubit coherence, scaling systems, and developing quantum software. However, hybrid approaches, combining quantum and classical systems, are gaining traction, leveraging the strengths of both for practical implementations.
Historical Context and Future Prospects
The journey of quantum computing began with theoretical foundations in the 1970s, gaining momentum with Feynman’s insights in 1982. By the 1990s, algorithms like Shor’s and Grover’s demonstrated potential speedups, leading to experimental systems in the 2000s. Recent years have seen rapid growth, with companies like IBM, Google, and startups like Rigetti and Quantinuum pushing boundaries. The 2024 Global Overview of Quantum Unicorn Enterprises report highlights nine quantum unicorns, primarily in the US and China, averaging 4.4 years to reach unicorn status, with valuations up to $3.67 billion.
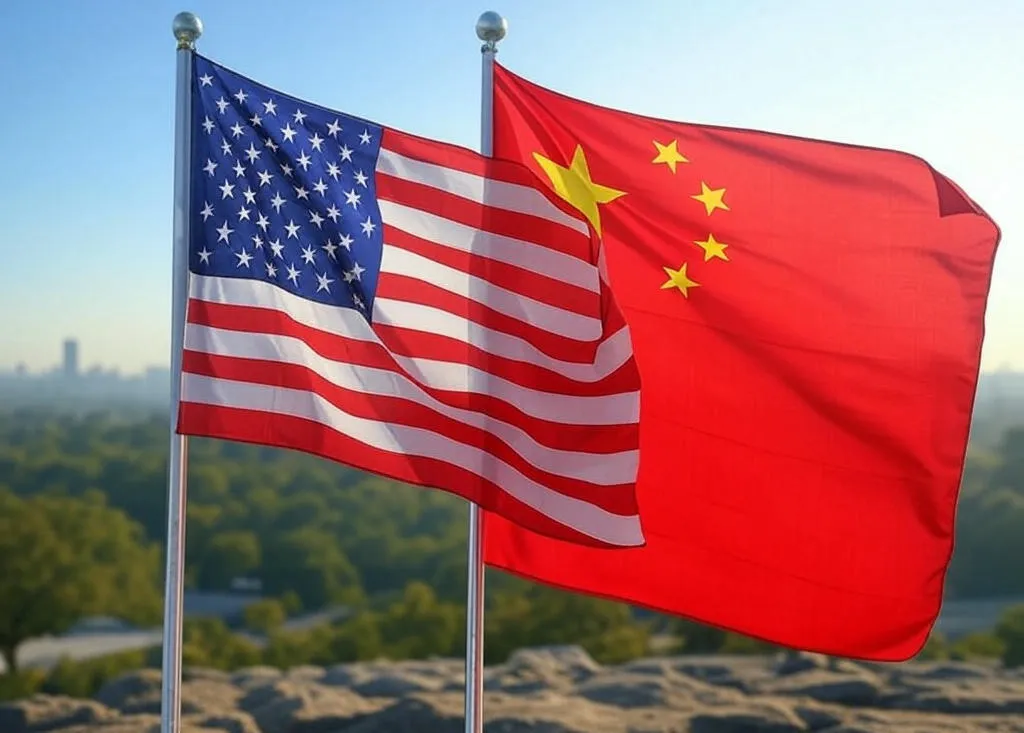
Looking ahead, 2025 is expected to bring breakthroughs in qubit fidelity, error correction, and practical applications, potentially achieving quantum advantage—where quantum computers consistently outperform classical ones for specific tasks. This could revolutionize industries, but also poses risks, such as breaking current encryption, necessitating quantum-resistant solutions.
Read more – Smart Nose leverages ML to detect food spoilage
Conclusion
Quantum computing in 2025 is at a critical juncture, with rapid advancements and global interest. It promises to solve problems beyond classical capabilities, from cryptography to material science, but remains in early development, facing significant technical challenges. The detailed understanding of its concepts, applications, and current state underscores its potential to reshape technology, with 2025 marking a pivotal year for progress.
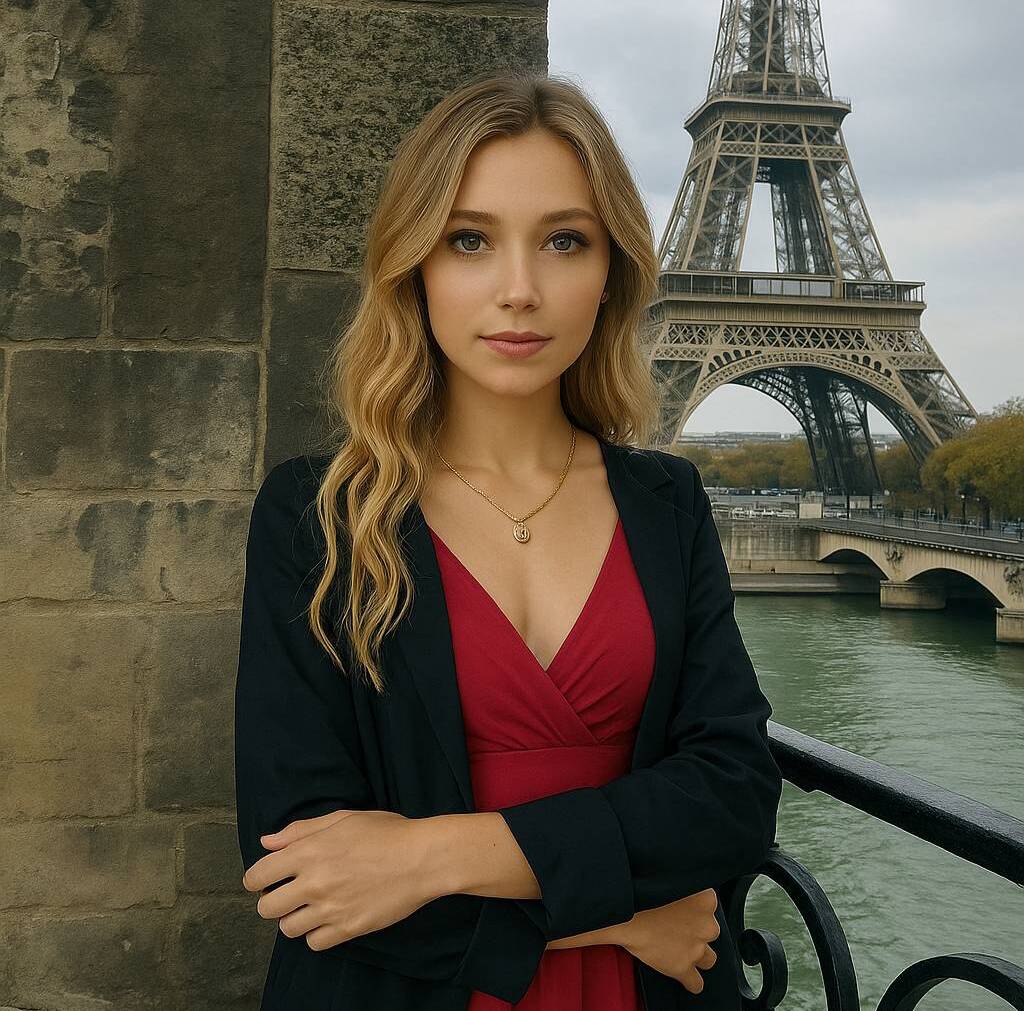
Passionate AI enthusiast and writer, I explore the latest advancements, trends, and ethical implications of artificial intelligence. Through my blog, I aim to simplify complex AI concepts and spark meaningful conversations about its impact on our future.